Magnetic Resonance Imaging (MRI) utilizes strong magnetic fields and radiofrequency pulses to generate detailed images of the body's internal structures. Hydrogen atoms in the body align with the magnetic field produced by a powerful magnet. Radiofrequency pulses then disturb this alignment, causing the hydrogen nuclei to emit signals as they return to their original state. These signals are captured by receiver coils and processed by advanced computer algorithms to construct highly detailed images. The process is non-invasive and does not involve radiation, making it a safer diagnostic tool. Exploration of these principles further reveals its extensive diagnostic capabilities.
MRI Highlights
- MRI uses powerful magnets to align hydrogen nuclei in the body and radiofrequency pulses to disturb their equilibrium.
- Protons emit signals as they relax back to alignment, which are detected by receiver coils.
- Magnetic field gradients are applied to encode spatial information, aiding in image construction.
- Complex algorithms process the collected data to produce detailed images of internal structures.
- MRI avoids radiation and surgical procedures, offering a safer and non-invasive diagnostic option.
Magnetic Resonance Imaging Basics
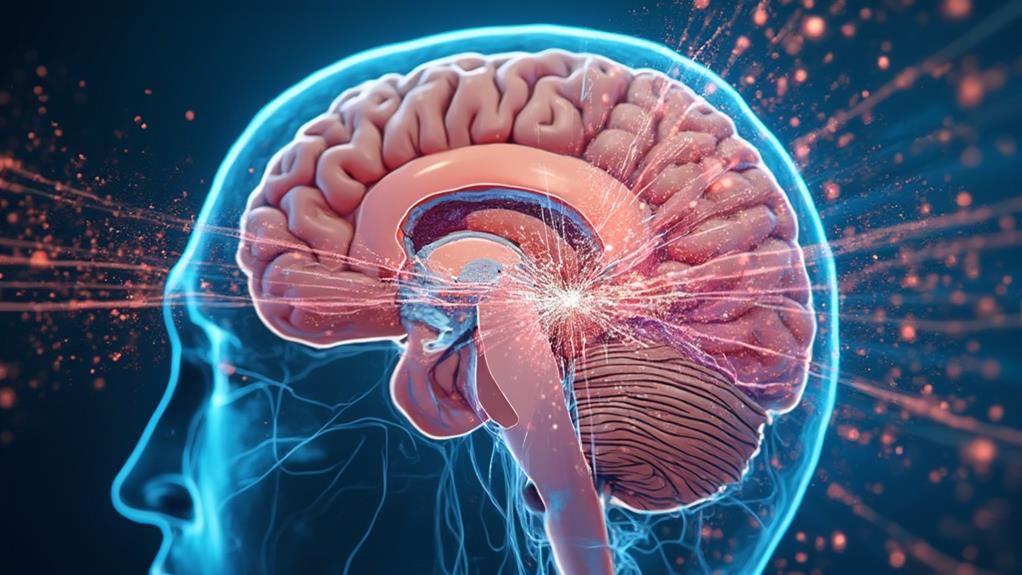
Magnetic Resonance Imaging (MRI) is based on the principles of nuclear magnetic resonance, which involves aligning the magnetic moments of hydrogen nuclei in the body using a powerful magnetic field. MRI is particular useful because it requires no radiation, making it a safer diagnostic option.
Key components of an MRI machine include the magnet, gradient coils, and radiofrequency coils, all working in harmony to facilitate image generation. The process of image generation involves transmitting radiofrequency pulses to disturb the alignment of hydrogen atoms, then measuring the emitted signals as these atoms realign, creating detailed cross-sectional images of the body's internal structures.
This noninvasive evaluation method allows for the detailed examination of the brain and other internal organs without the need for surgery.
Underlying Physical Principles
At the heart of MRI technology lies the fundamental principle of nuclear magnetic resonance. This phenomenon occurs when nuclei in a strong magnetic field absorb and re-emit electromagnetic radiation. The principle is rooted in the magnetic properties of atomic nuclei, particularly hydrogen nuclei, which are abundant in human tissues due to the high water content.
When placed in a magnetic field, these hydrogen nuclei align with the direction of the field. By applying a radiofrequency pulse, the nuclei are temporarily disoriented, causing them to emit signals as they realign with the magnetic field. These emitted signals are captured and recorded by the MRI system. The variations in the emitted signals provide a detailed map of different tissue types and structures within the body.
The resonance frequency of the hydrogen nuclei depends on the strength of the magnetic field, enabling precise localization and imaging of specific body areas. The differential relaxation times of tissues, as hydrogen nuclei return to their original state, further refine the imaging quality. Through complex algorithms, these signal differences are converted into highly detailed visual representations, ultimately aiding healthcare professionals in diagnosing and treating various medical conditions.
Core Components Explained
Understanding the brainwork behind MRI technology requires dissecting its core components. At the heart of an MRI system is a powerful magnet, typically a superconducting magnet cooled to cryogenic temperatures using liquid helium. This magnet generates a strong, stable magnetic field, often measured in teslas, which aligns the protons in the body.
Next, MRI machines utilize gradient coils to produce varying magnetic fields. These coils are essential for spatial encoding of the MRI signal, allowing the machine to localize the origin of signals from specific body parts. The gradient coils operate along three axes—X, Y, and Z—enabling detailed imaging in multiple dimensions.
Another key component is the radiofrequency (RF) system, comprising coils that transmit and receive RF signals. The RF transmitter coil sends pulses that disturb the aligned protons, prompting them to emit signals captured by the RF receiver coil. These signals are then processed to construct images.
Further, the system includes a computer with advanced software to control the hardware components and analyze the received data. This computer interprets the signals and converts them into understandable visual images, which radiologists can then examine for diagnostic purposes. Together, these components form the backbone of MRI technology.
Image Generation Process
The process of generating images through MRI technology hinges on a detailed interplay of magnetic fields and radiofrequency pulses. At the core, MRI scans exploit the magnetic properties of hydrogen atoms abundant in the human body.
When a patient enters the MRI machine, a powerful magnet aligns the proton spins within these hydrogen atoms. Following this alignment, the machine emits precise radiofrequency pulses to disturb this equilibrium momentarily.
Once the radiofrequency pulse is turned off, the protons relax back to their original state, emitting signals in the process. These emitted signals are detected by the MRI machine's receiver coils. By adjusting various parameters such as pulse sequences and magnetic field gradients, the MRI system can target specific tissues, enhancing the contrast between different types of biological matter.
The collected data is processed using complex algorithms, resulting in the detailed images known for helping in the diagnosis of a wide range of conditions. Each image slice can be constructed into an extensive three-dimensional representation, providing clinicians with invaluable insights. This meticulous process illustrates how MRI technology serves healthcare providers, enhancing their ability to deliver patient care with precision and clarity.
Benefits
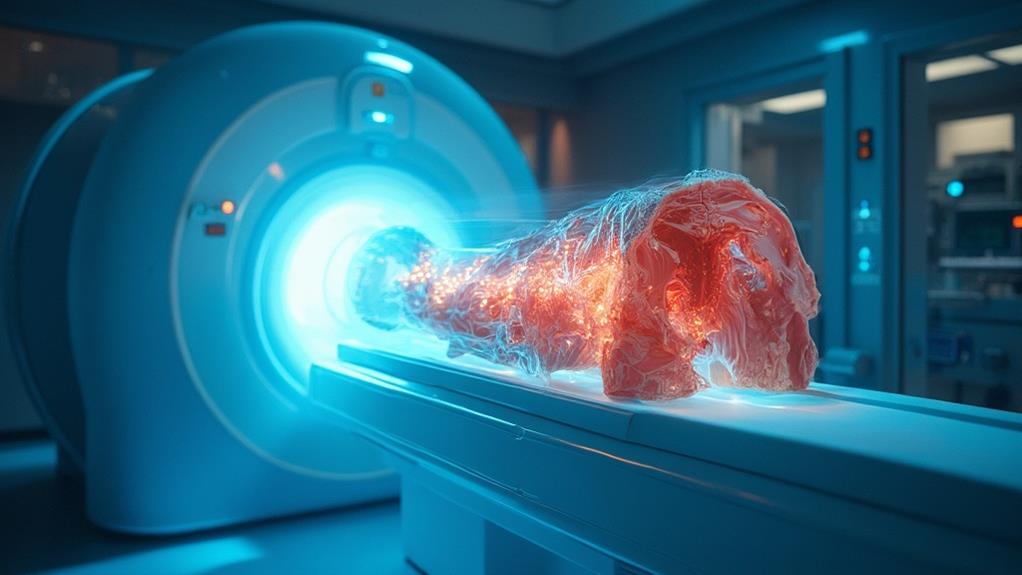
MRI scans offer numerous benefits, including being a non-invasive diagnostic tool that provides detailed imaging of the body's internal structures. These scans produce clearer and better diagnostic images compared to other modalities and are especially helpful in evaluating symptoms like vertigo, headaches, seizures, or vision issues.
Additionally, unlike other imaging techniques that use ionizing radiation, MRI scans pose no radiation exposure risk to patients. These features make MRI a valuable tool in detecting diseases at an early stage, which is essential for timely treatment.
Non-Invasive Diagnostic Tool
One significant benefit of MRI scans as a non-invasive diagnostic tool is their ability to produce detailed images of the body's internal structures without requiring surgical procedures or exposure to ionizing radiation. This capability makes MRI an excellent option for many patients, particularly those for whom surgical risks must be minimized or who might be vulnerable to the harmful effects of radiation, such as pregnant women and children.
MRI technology utilizes strong magnetic fields and radio waves, generating extensive images through a non-intrusive process. Patients experience no discomfort, and the procedure can be repeated as needed, providing continuous monitoring over time without added risk. This non-invasive nature eliminates the need for recovery periods associated with surgical diagnosis, resulting in less strain on healthcare services and reducing patient downtime.
Furthermore, MRI scans can be pivotal in detecting and managing numerous conditions, from neurological disorders to musculoskeletal injuries and internal organ diseases. The non-invasive approach fosters detailed and timely diagnostics, enabling healthcare providers to craft precise treatment plans, thereby enhancing patient care and outcomes. This efficiency underscores the importance of MRI scans in contemporary medical practice, reinforcing their value in promoting patient well-being without compromising safety.
Detailed Body Imaging
Building on the advantages of its non-invasiveness, another prominent benefit of MRI technology lies in its ability to deliver exceptionally detailed body imaging. MRI scans produce high-resolution images that allow healthcare providers to examine the body's internal structures with remarkable clarity. These images are generated using powerful magnetic fields and radio waves, which interact with hydrogen atoms in the body, producing detailed cross-sectional images.
This level of precision makes MRI particularly valuable for visualizing soft tissues, such as the brain, muscles, ligaments, and heart. Unlike other imaging methods, MRI does not rely on ionizing radiation, which further enhances its safety profile while maintaining diagnostic accuracy. By providing clear images of intricate bodily regions, MRI supports healthcare professionals in making accurate diagnoses and formulating appropriate treatment plans.
Moreover, the extensive imaging capabilities of MRI scans can aid in the monitoring of existing conditions, tracking changes and ensuring that interventions are effective. This guarantees that patients receive the most appropriate and timely care. In sum, detailed body imaging through MRI substantially enhances the ability of doctors and medical practitioners to serve the health needs of individuals with precision and care.
Early Disease Detection
A significant benefit of MRI technology is its role in the early detection of diseases, which can substantially improve patient outcomes. MRI scans provide highly detailed images of soft tissues, organs, and other internal structures, enabling clinicians to diagnose conditions at stages when they are more treatable. This capability is particularly essential for diseases like cancer, where early detection can lead to more effective and less invasive treatment options.
In addition to malignancies, MRI technology is instrumental in identifying neurological disorders, cardiovascular abnormalities, and musculoskeletal conditions. For instance, early diagnosis of multiple sclerosis or Parkinson's disease through MRI can allow for timely intervention, potentially slowing the disease's progression and enhancing the patient's quality of life. Moreover, MRI scans can detect small anomalies in the cardiovascular system, such as plaques or vessel wall thickening, alerting physicians to potential risks before they result in severe events like heart attacks or strokes.
No Radiation Exposure
Unlike imaging techniques that rely on ionizing radiation, such as X-rays and CT scans, MRI technology offers the significant benefit of posing no radiation exposure to patients. This characteristic is particularly advantageous for individuals requiring multiple scans or those with conditions necessitating routine monitoring.
The benefits of zero radiation exposure through MRI scans are substantial:
- Safety for Vulnerable Populations: Children, pregnant women, and individuals with chronic illnesses can undergo MRI scans without the risks associated with ionizing radiation.
- Repetitive Use: Patients needing repeated imaging, such as cancer survivors or those with chronic diseases, can safely have multiple MRI scans without cumulative radiation risks.
- Prolonged Health Monitoring: Regular MRI scans provide safe long-term monitoring of certain conditions, facilitating early detection and effective management without added radiation exposure.
- Compatibility with Diverse Conditions: MRIs can be used for a wide range of diagnostic purposes, from brain and spinal cord investigations to joint and soft tissue evaluations, offering a versatile tool without radiative concerns.
Contrast Dye Usage
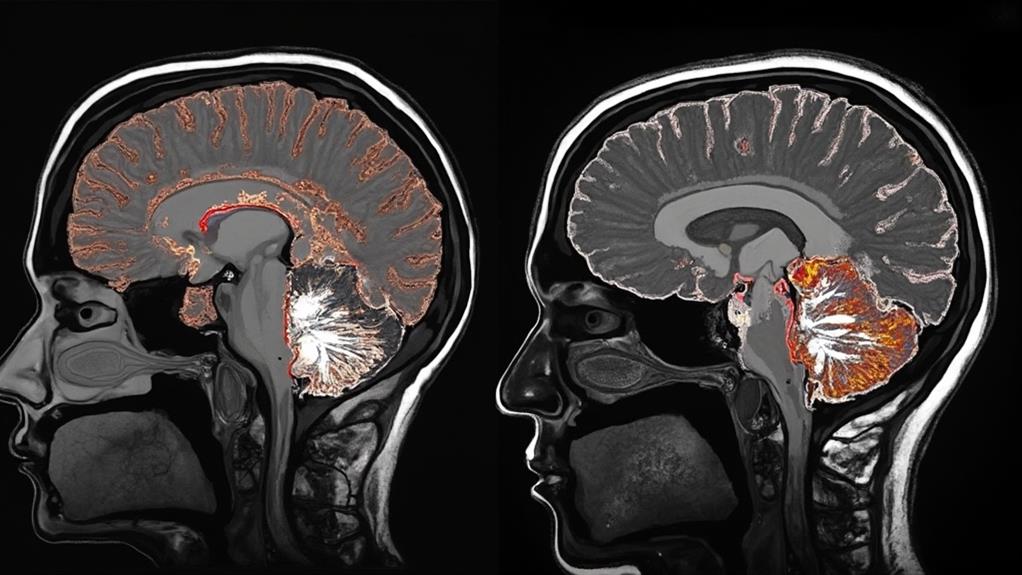
Contrast dyes play a critical role in enhancing the visibility of specific tissues and blood vessels during MRI scans, improving diagnostic accuracy. These dyes, primarily gadolinium-based, are carefully administered to guarantee patient safety while providing detailed imaging. Below is a comparison of different types of contrast dyes and their key characteristics:
Type | Composition | Usage |
---|---|---|
Gadolinium-based | Metal ion | Highlight blood vessels |
Iron-based | Superparamagnetic | Detect inflammation |
Manganese-based | Paramagnetic ion | Liver imaging |
Understanding these types aids in selecting the appropriate contrast agent for various diagnostic requirements.
Types of Contrast Dyes
Various types of contrast dyes exist to enhance the diagnostic capabilities of MRI scans. These dyes, known as contrast agents, help to improve the clarity and detail of the images produced, making it easier to identify abnormalities and plan treatments. The choice of contrast dye depends on several factors, including the patient's medical history and the specific diagnostic requirements.
- Gadolinium-Based Agents: These are the most commonly used MRI contrast agents. Gadolinium enhances the visibility of blood vessels, tumors, and inflammation. It is generally safe but requires caution in patients with kidney issues.
- Iron Oxide Nanoparticles: These agents are utilized primarily in liver imaging. They provide a high degree of contrast and are particularly useful for identifying liver lesions and tumors.
- Manganese-Based Agents: Useful in liver and pancreas imaging, manganese-based contrast agents offer clear images of these specific organs. They complement gadolinium-based agents in specialized applications.
- Perflubron-Based Agents: These agents are currently in development and show promise in lung imaging. They potentially offer a new avenue for diagnosing respiratory conditions, although their usage is not yet widespread.
In choosing the appropriate contrast dye, healthcare providers aim to offer the most accurate diagnoses while considering patient-specific factors to guarantee safety and efficacy.
Importance of Contrast Dyes
The significance of contrast dyes in MRI scans cannot be overstated, as these substances play an indispensable role in enhancing the quality of the diagnostic images obtained. Contrast dyes, often gadolinium-based, are injected to improve the visibility of internal structures. By altering the magnetic properties of nearby hydrogen atoms in water molecules, contrast dyes amplify the difference between normal and abnormal tissues.
For medical professionals aiming to provide the best care, contrast dyes offer an invaluable tool. They make it easier to differentiate between healthy and diseased tissue, diagnose conditions like tumors, inflammation, and infections, and assess vascular abnormalities. The enhanced clarity helps radiologists identify issues that might be invisible on standard MRI scans.
The benefits of contrast dyes extend beyond mere visibility. They offer precise visualization of blood vessels and tissues, aiding in accurate mapping before surgical procedures. For patients, this means a higher likelihood of accurate diagnoses, effective treatment plans, and improved outcomes. In other words, contrast dyes play a fundamental role in modern medicine, enabling healthcare providers to deliver high-quality, detailed diagnostic images that facilitate better patient care and support informed medical decisions.
Administration and Safety
While the benefits of contrast dyes in MRI scans are clear, their effective administration and safety considerations are paramount. Safeguarding the responsible use of these agents is fundamental to providing accurate diagnostic information while protecting patient health.
Pre-Screening: Before administering contrast dye, thorough patient evaluation is essential. Reviewing medical histories, particularly renal function and allergies, helps mitigate potential adverse reactions.
Dosage and Administration: The correct dosage of contrast dye based on patient weight and medical condition is pivotal. This precision minimizes risk and optimizes imaging results. Intravenous injection is commonly employed, guaranteeing timely dye dispersion.
Monitoring Patient Response: Continuous monitoring during and after the MRI scan is crucial. Watching for any signs of anaphylactic reactions or other side effects allows for prompt intervention. Technicians and radiologists must be trained to handle emergencies.
Gadolinium Concerns: Being mindful of the risks associated with gadolinium-based contrast agents, particularly in patients with kidney issues, is indispensable. Implementing protocols for using safer alternatives when necessary safeguards patient safety.
MRI FAQ
How Loud Is the MRI Machine During a Scan?
MRI machines can be quite loud, reaching noise levels between 70 to 110 decibels. To guarantee the comfort and well-being of patients, professionals often provide earplugs or noise-canceling headphones during the scan.
Can You Move During an MRI Scan?
During an MRI scan, it is imperative to remain still to guarantee the accuracy of the images. Movement can result in blurred images, which can lead to misinterpretation and potentially affect the quality of patient care.
Are There Any Side Effects After an MRI Scan?
While MRI scans are generally safe, some patients may experience temporary side effects such as dizziness, headache, or mild nausea. Communicating efficiently with your patients can alleviate concerns and guarantee a more comfortable experience for them.
How Long Does an MRI Scan Typically Take?
An MRI scan typically takes between 30 to 60 minutes, depending on the specific areas being examined and the complexity of the study. Offering thorough care, we guarantee patients remain comfortable and informed throughout the procedure.
Can You Bring Personal Items Into the MRI Room?
Patients are advised to avoid bringing personal items into the MRI room, as the strong magnetic field can interfere with electronic devices and attract metallic objects. Removing such items guarantees safety and prevents potential damage to equipment.