Functional MRI (fMRI) is a cutting-edge, non-invasive imaging technique essential for studying brain activity. By tracking changes in blood flow and oxygenation levels, fMRI can identify regions of the brain active during specific tasks or experiences. The technique relies on the body's response to magnetic fields, particularly the behavior of hydrogen atoms subjected to strong magnetic forces and radiofrequency pulses. While fMRI offers high spatial resolution and valuable insights into neural networks, it faces challenges such as data variability, signal artifacts, and limited temporal resolution. Exploring these aspects can uncover deeper understandings of fMRI and its applications.
MRI Highlights
- Functional MRI (fMRI) measures brain activity by detecting changes in blood flow related to neural activity.
- fMRI uses BOLD (blood oxygen level-dependent) contrast to identify active brain regions.
- The technique is non-invasive, allowing for safe and repeated brain imaging.
- High spatial resolution allows detailed visualization of brain activity, aiding in understanding neural networks.
- fMRI faces challenges like signal artifacts, statistical thresholding, and temporal resolution limitations.
How Fmri Works
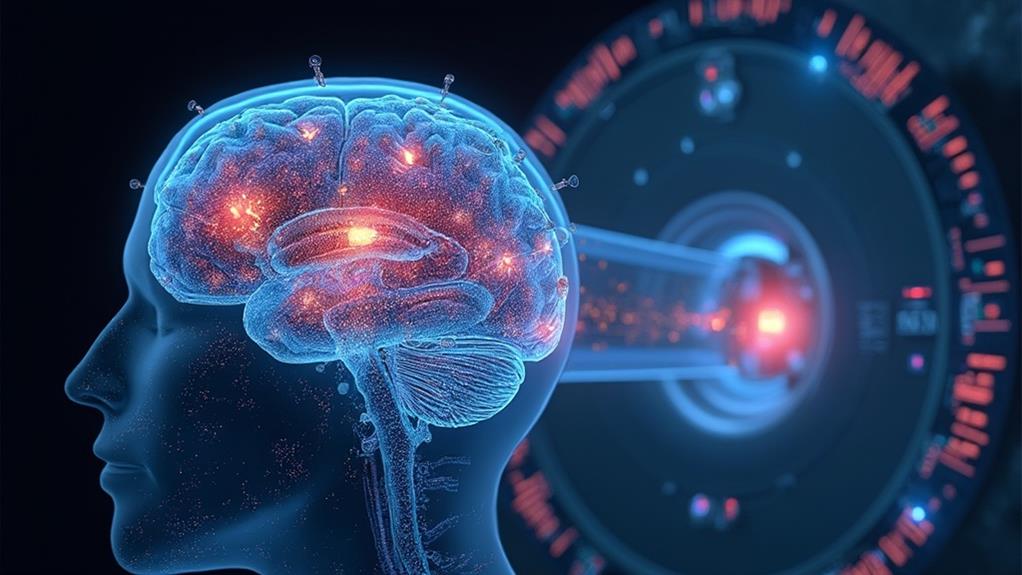
Functional MRI (fMRI) measures brain activity by detecting changes in blood oxygen levels, leveraging the fact that oxygen-rich and oxygen-poor blood respond differently to magnetic fields. When a specific region of the brain is more active, it consumes more oxygen, resulting in increased blood flow to that area.
This oxygenation change alters the magnetic resonance signal, allowing researchers to map functional activity within the brain. Similar to the vascular MRI, fMRI provides non-invasive inspection of tissues, offering detailed information essential for understanding brain function.
Brain Activity Measurement
Often regarded as a revolutionary tool in neuroscience, fMRI measures brain activity by detecting changes in blood flow, a process known as hemodynamic response. This technique is predicated on the principle that neural activity and cerebral blood flow are intrinsically linked.
When a specific region of the brain is activated, it demands more oxygen. This heightened demand results in increased blood flow to the area, facilitating the fMRI to capture these variations.
The process begins by placing the subject within an MRI scanner, which generates a strong magnetic field. Radiofrequency pulses are then employed to disturb the alignment of hydrogen atoms in the brain. As these atoms realign, they emit signals that are detected and translated into images by a computer. These images reveal the precise locations of brain activity during various tasks or at rest.
fMRI's non-invasive nature is one of its most compelling attributes, allowing researchers and clinicians to observe and measure brain function without inflicting harm or discomfort. By providing real-time, dynamic insights into brain activity, fMRI has become a pivotal instrument for understanding complex neural processes and advancing our knowledge in fields such as cognitive neuroscience, psychology, and neurology.
Blood Oxygen Levels
The effectiveness of fMRI hinges on its ability to assess blood oxygen levels in the brain. This capability is indispensable as it enables the identification of brain regions that are active during specific tasks or experiences. Active neurons consume oxygen at a higher rate, leading to localized changes in blood oxygenation. To supply these neurons with sufficient oxygen, the body compensates by increasing blood flow. This change in blood oxygenation can be detected by fMRI.
The underlying principle is based on the Blood Oxygen Level Dependent (BOLD) contrast. BOLD signals are sensitive to the differences in oxygenated and deoxygenated hemoglobin, which have distinct magnetic properties. When neural activation occurs, the increase in oxygenated blood outpaces the increase in deoxygenated blood, resulting in a higher BOLD signal. This allows researchers to map areas of neural activity with considerable spatial resolution.
fMRI provides invaluable insights for those aiming to understand and alleviate neurological conditions, enhance therapeutic approaches, and tailor interventions for ideal patient care. By interpreting these blood oxygen level fluctuations, professionals can better comprehend brain function and improve strategies for cognitive rehabilitation, mental health treatments, and a range of other applications essential to healthcare.
Magnetic Field Response**
At its core, fMRI operates by capitalizing on the body's response to magnetic fields, providing detailed insights into brain activity. This technique leverages the inherent magnetic properties of hydrogen atoms, which are abundant in water molecules throughout the body. When the subject is placed in a high-strength magnetic field, these hydrogen protons align with the direction of the field.
An fMRI scanner emits radiofrequency pulses that temporarily disrupt this alignment. As the protons return to their original aligned state, they emit signals that the scanner detects and processes. This process hinges on the differing magnetic properties of oxygenated and deoxygenated blood. Oxygenated blood is diamagnetic, whereas deoxygenated blood is paramagnetic. These distinct properties affect the local magnetic field in brain regions, altering the emitted signals.
Changes in blood flow associated with neural activity lead to variations in the local concentration of deoxygenated hemoglobin. The fMRI identifies these changes, known as the Blood Oxygen Level Dependent (BOLD) signal. Through complex algorithms, this data is converted into detailed images depicting active brain regions. This non-invasive method provides critical insights, helping professionals deliver better care and facilitating research that can benefit countless individuals.
Benefits
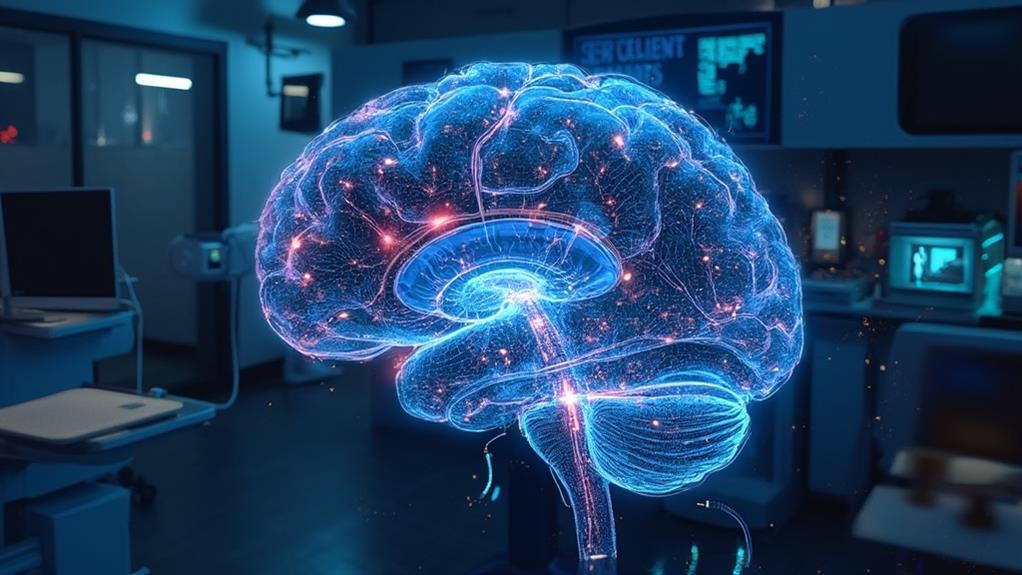
Functional MRI (fMRI) offers several key benefits that make it an invaluable tool in neuroscience and clinical practice. As a non-invasive imaging technique, it allows for the detailed visualization of brain structures with high spatial resolution while providing insights into real-time brain activity.
This capability to monitor cognitive function in real-time facilitates a deeper understanding of neurophysiological processes and aids in the diagnosis and treatment of neurological disorders. Additionally, fMRI uses strong magnetic fields and radio waves, similar to the high-field MRI provided by some affordable MRI services, consequently ensuring superior imaging quality.
Non-invasive Brain Imaging
Non-invasive brain imaging techniques, particularly functional MRI (fMRI), offer a multitude of benefits by allowing researchers and clinicians to observe brain activity without the need for surgical procedures. This non-invasive nature reduces risk to patients, eliminating complications associated with invasive methods such as infection or adverse reactions to anesthesia. As a result, fMRI makes it possible to study brain function in a broad demographic, including vulnerable populations such as children or individuals with severe medical conditions who might otherwise be excluded from research.
The ability to repeatedly conduct fMRI scans on the same individual enables longitudinal studies, observing changes in brain activity over time. This is particularly important for tracking the progression of neurological diseases or the impact of therapeutic interventions. Additionally, fMRI's non-invasive nature improves patient comfort, which can enhance compliance and the overall quality of data collected.
Non-invasive imaging also plays a critical role in the preoperative planning for neurosurgical procedures. By mapping critical brain areas, surgeons can strategize to avoid these regions, preserving essential functions such as speech or motor skills. Moreover, because fMRI does not involve exposure to ionizing radiation, it is safer for repeated use, making it a practical tool for continuous monitoring and follow-up.
High Spatial Resolution
One of the primary advantages of functional MRI is its high spatial resolution, enabling detailed visualization of brain activity at the millimeter scale. This capability allows researchers and clinicians to identify specific regions of the brain that are activated during various cognitive tasks and behaviors. Such precision is invaluable for understanding the complex neural networks and for diagnosing and treating neurological disorders.
High spatial resolution in fMRI offers numerous benefits, particularly for those in fields centered on aiding others through healthcare and scientific research. These benefits include:
- Enhanced Diagnostic Accuracy: Fine spatial detail improves the ability to detect and diagnose subtle brain abnormalities, aiding early intervention and treatment planning.
- Targeted Therapeutic Interventions: Pinpointing specific brain regions involved in certain functions can inform more precise therapeutic approaches, including targeted brain stimulation or surgical interventions.
- Improved Understanding of Brain Function: Detailed maps of brain activity enable a deeper understanding of the relationship between various brain regions and their functions, contributing to more effective treatments and interventions.
- Advancement in Brain Research: High-resolution imaging supports groundbreaking research endeavors, facilitating the discovery of novel insights into brain structure and function, ultimately enhancing patient care.
These advantages underscore the profound impact of high spatial resolution fMRI on improving the efficacy of brain-related medical and research efforts.
Real-time Brain Activity
The ability to monitor real-time brain activity using fMRI has revolutionized the field of neuroscience and clinical practice. This technological advancement provides unparalleled benefits, allowing researchers and clinicians to observe and interpret brain functions as they occur. Real-time fMRI offers immediate feedback on neural activity, enabling interventions for improving cognitive functions and treating neurological disorders to be more accurately tailored.
Furthermore, real-time brain activity monitoring facilitates the development of neurofeedback therapies aimed at treating conditions such as anxiety, depression, and ADHD. By watching their own brain activity patterns in real-time, patients can learn to regulate their neural responses, promoting mental well-being and enhancing cognitive control. This personalized approach guarantees that interventions can be adjusted instantly, maximizing therapeutic effectiveness.
Additionally, real-time fMRI supports research into the neural underpinnings of behavior, providing insights that are crucial for developing new treatments. It allows for the rapid identification of brain regions involved in specific tasks, accelerating the understanding of complex neural networks. Overall, the ability to monitor real-time brain activity enhances both our comprehension of the brain and our capacity to serve patients more effectively, leading to improved clinical outcomes and patient care.
Cognitive Function Insights
A growing body of research utilizing fMRI has provided profound insights into the workings of cognitive functions, greatly benefiting both scientific understanding and clinical applications. This non-invasive technique measures brain activity by detecting changes in blood flow, allowing researchers to investigate how different areas of the brain contribute to various cognitive processes.
These insights have several notable benefits:
- Enhanced understanding of brain networks: fMRI has revealed complex networks within the brain, such as the default mode network, which is active during rest and introspection. This knowledge aids in identifying how different brain regions interact functionally.
- Improved diagnosis of neurological disorders: By observing abnormal brain activity patterns, fMRI can help diagnose conditions like Alzheimer's disease, schizophrenia, and depression, enabling earlier intervention and more targeted treatments.
- Informing rehabilitation strategies: For patients recovering from strokes or traumatic brain injuries, fMRI data can guide the development of personalized rehabilitation plans, enhancing recovery outcomes.
- Advancing educational techniques: Insights into how the brain processes information can inform teaching methods, potentially leading to improved educational outcomes for diverse learning populations.
Limitations and Challenges
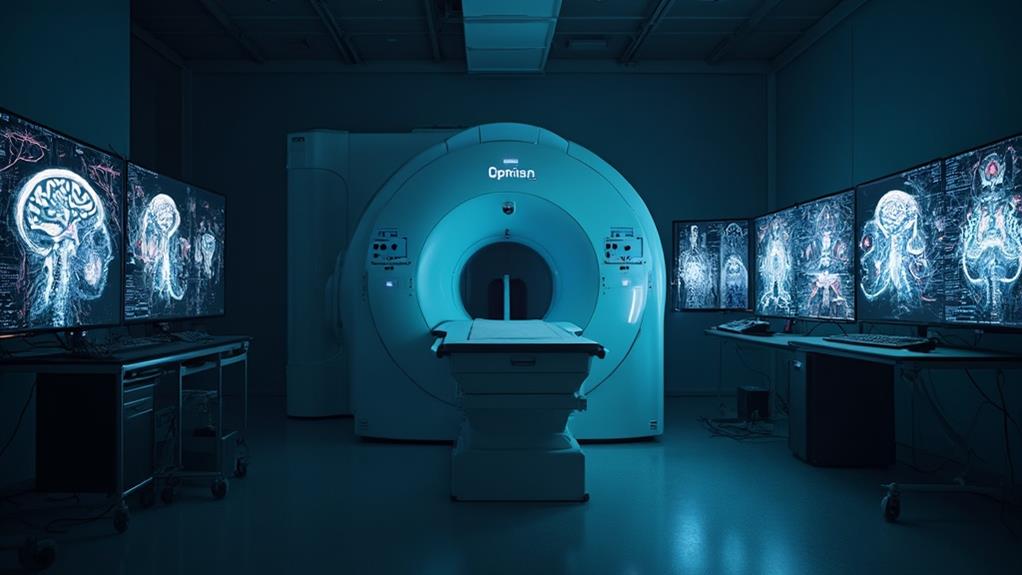
Despite its numerous benefits, fMRI is not without its limitations and challenges. The technology faces constraints related to spatial and temporal resolution, as well as issues with data interpretation, all of which can affect the accuracy and efficacy of results. The table below summarizes these key challenges:
Limitation | Description |
---|---|
Spatial Resolution | Difficulty in pinpointing exact brain locations. |
Temporal Resolution | Inability to capture rapid brain activities accurately. |
Data Interpretation | Complex and sometimes ambiguous data analysis. |
These factors must be addressed to optimize fMRI's utility in research and clinical settings.
Spatial Resolution Constraints
Many factors constrain the spatial resolution of functional MRI (fMRI), posing substantial challenges for researchers and clinicians alike. Achieving high spatial resolution is essential for accurately mapping brain activity and understanding neurological processes. However, several limitations impede obtaining finer details.
- Signal-to-Noise Ratio (SNR): Higher spatial resolution requires smaller voxel sizes, which can reduce the SNR, making it more difficult to distinguish relevant brain activity from background noise.
- Magnetic Field Strength: While higher magnetic field strengths (e.g., 7 Tesla and above) can enhance spatial resolution, they also introduce increased susceptibility artifacts and other technical complications.
- Head Movement: Patient movement during scanning can blur images, drastically affecting spatial resolution. Even minor involuntary movements can distort results.
- Imaging Speed: To capture higher spatial resolution, longer acquisition times are often necessary, which may not be practical in clinical settings due to patient discomfort and time constraints.
Balancing these constraints requires meticulous optimization of scanning parameters and techniques, often involving trade-offs between spatial resolution, temporal resolution, and patient comfort. The quest for enhanced spatial resolution in fMRI continues to evolve, driven by technological advancements and innovative methodologies, ultimately aiming to better serve clinical diagnoses and research objectives.
Temporal Resolution Limitations
Understanding temporal resolution limitations in functional MRI (fMRI) is essential for interpreting dynamic brain activities. Temporal resolution refers to how frequently images can be captured to observe changes over time. In fMRI, this is constrained by the hemodynamic response, which typically lags neuronal activity by a few seconds. Because fMRI captures data based on changes in blood oxygenation, the temporal resolution is inherently slower compared to direct neural recording methods like electroencephalography (EEG).
The standard temporal resolution for fMRI is in the range of one to two seconds per image, which may not adequately capture rapid neuronal events occurring at the millisecond level. Consequently, this limitation affects the ability to observe fast cognitive processes and intricate temporal dynamics of brain function. Researchers must therefore carefully design their experiments, often employing block designs or event-related designs, to mitigate some of these constraints.
While advanced techniques such as multiband imaging have improved temporal resolution, the trade-off with signal-to-noise ratio and spatial resolution must be considered. In serving others, it remains important to balance these factors to obtain the most accurate and meaningful insights into brain function dynamics.
Data Interpretation Issues
Interpreting data from functional MRI (fMRI) presents a range of limitations and challenges that must be meticulously addressed to guarantee the accuracy of conclusions drawn. One significant issue is the indirect nature of the fMRI signal, which is based on blood oxygen level-dependent (BOLD) contrasts rather than neural activity itself. This can result in ambiguities when linking hemodynamic responses to specific neural events.
Moreover, the spatial resolution of fMRI, though relatively high compared to other imaging techniques, remains limited in distinguishing fine-grained neural structures, leading to potential misinterpretations of where brain activity genuinely occurs.
Additional challenges include:
- Signal Artifacts: Variability in signal caused by head movement, physiological noise, and scanner-specific anomalies.
- Statistical Thresholding: Determining appropriate thresholds for significance to avoid false positives, which can lead to erroneous findings.
- Temporal Resolution: fMRI typically offers lower temporal resolution, making it difficult to capture rapid neural dynamics and discriminate between closely timed events.
- Inter-individual Variability: Differences in brain anatomy and function across individuals can complicate the standardization and generalization of results.
Addressing these issues requires careful experimental design, appropriate statistical methods, and multi-modal approaches integrating data from complementary neuroimaging techniques, ensuring robust and reliable interpretations that advance human well-being.
MRI FAQ
What Medical Conditions Can Be Diagnosed Using Fmri?
The medical conditions that can be diagnosed include neurological disorders such as Alzheimer's, Parkinson's disease, epilepsy, and multiple sclerosis. It enables early detection and precise monitoring, enhancing care for patients with intricate neurological needs.
How Long Does a Typical Fmri Scan Take?
A typical scan generally ranges from 30 to 60 minutes, depending on the specific protocols used and the areas being examined. This detailed imaging process allows healthcare professionals to accurately assess and serve patients' neurological needs.
Is Fmri Safe for All Age Groups?
Magnetic resonance imaging (MRI) is generally considered safe for all age groups, provided there are no contraindications such as implanted medical devices or metal fragments. Medical professionals must assess individual patient history to guarantee safety.
How Should a Patient Prepare for an Fmri Scan?
Prior to a scan, patients should inform their healthcare provider of any metal implants or medical devices. Remove all jewelry and metal objects, and wear comfortable clothing. Follow specific pre-scan instructions to guarantee accurate results.
Can Fmri Detect Mental Health Disorders?
Detection of mental health disorders should encompass various diagnostic tools and clinical assessments. While certain brain patterns can correlate with mental health conditions, relying solely on such imaging for conclusive diagnoses is not yet a standard practice.